Introduction
Our everyday experience suggests that we have a vivid, almost photographic understanding of the world around us through visual perception. The belief is a simplified view of a complex process. Visual insight is the brain’s capability to understand and make sense of visual data received from our eyes, linking the recognition of shapes, colours, depth, and spatial relationships between objects. Todorović (2020) conducted a study to prove how optical illusions, such as the Müller-Lyer illusion, can create an apparent reality that may not align with the actual physical measurement. Similarly, Ismail and Paravina (2021) discuss the idea of colour adjustment potential in resin composites, raising fascinating questions about whether it is primarily an optical illusion. Through these comprehensive studies, it is evident that optical illusions play a significant role. Divergent to the common belief that our visual insight provides a richly comprehensive, almost photographic depiction of the world. The essay contends that our perception is a complex interaction of physiological, cognitive, and neurological processes that regularly misrepresents the true nature of our visual environment.
The Nature of Visual Perception
National Eye Institute (2019) states that vision is a complex and fascinating process that begins when light enters the eye, a vital step in perceiving the world around us. The cornea, a transparent dome-shaped layer, is the eye’s first point of contact with light. Its curved structure refracts the light, starting the process of focusing (See Fig 1). The light then travels through the pupil, the central opening in the iris, which is the coloured part of the eye. The iris functions like a camera shutter, expanding or contracting to regulate the amount of light entering the eye. Light meets the lens past the pupil, a clear, flexible structure that further corrects focus. The fine-tuning warrants that light rays precisely converge on the retina, the light-sensitive layer lining the back of the eye. The photoreceptor cells, known as rods and cones, perform a vital transformation. They convert light into electrical signals, a language the brain understands. Rods are adept at sensing low light levels and are critical to our night vision, while cones are in charge of colour discernment and detail in well-lit conditions. These signals travel along the optic nerve to the brain, which interprets them to form the images one sees. The complex contact between the eyes, optic nerves, and brain allows us to perceive shapes, colours, and movement, incredibly inducing our interaction with and understanding of the world.
Fig 1. The Eye (National Eye Institute, 2019)
Limitations of Human Vision
Human vision, although remarkably capable, is subject to several inherent limitations because of the structure and function of the eye. The human retina is lined with two types of photoreceptors: cones and rods. Cones, numbering between 6 to 8 million, are focused in the central part of the retina (the fovea) and are highly sensitive to colour. The measure of the eye’s resolving power differs among individuals and is frequently assessed using a Snellen chart at an optometrist’s office. At a standard test distance, the width of the strokes of letters in the 20/20 row subtends an angle of 1 minute of arc (John Wiley & Sons, 2011). Normal vision is the ability to discern this row, but visual acuity can range from 0.5 to 5 minutes of arc, signifying substantial variation among people. These limitations highlight the complex interplay between the physical structure of the eye and the brain’s processing abilities, which shape our perception of the world.
Additionally, Deubel et al.’s (1996) study sheds light on the limitations of human vision, precisely in the context of saccadic eye movements and target displacement detection. The results highlight our visual system’s inherent challenges when tracking objects during rapid eye movements. The left-hand graph reveals that detection performance is suboptimal when the target remains displayed but is shifted during the saccade. It proposes that our visual system struggles to accurately perceive changes in an object’s position during the quick and jerky movements of the eye. Furthermore, the right-hand graph presents an intriguing aspect of human visual limitations. When the target is offset at the onset of the saccade and represented 250 milliseconds after the eyes have landed, detection performance significantly improves. This highlights the need for a momentary pause or stabilisation period for our visual system to process and identify environmental changes accurately. These articles highlight how vision is constrained by the mechanics of our eye movements and the necessity for certain temporal and spatial constraints for adequate perception.
Return of an accurate spatial code (Deubel et al. (1996 )
Evidence from Optical Illusions
Optical illusions are essential in shaping perception, indicating how visual stimuli can manipulate and distort understanding of the surrounding world. As discussed in the article by Eneh and Inekwe (2023), optical illusions offer fascinating insights into how the brain interprets and sometimes misinterprets visual information. For example, the Zollner illusion, where an image’s background interferes with the image’s perception, illustrates how visual context can influence perception. The illusion can even induce sensations like nausea, demonstrating the profound impact of visual cues on physical experience. The Muller-Lyer illusion, where two parallel lines appear unequal due to arrowheads, underscores how simple visual elements can dramatically change the perception of size and length. This illusion highlights the brain’s tendency to be influenced by surrounding visual cues. Equally, The Feature Integration Theory (FIT), as delineated by Kristjánsson and Egeth (2020), offers a valuable outline for understanding how optical illusions can occur. The FIT perceptual process is hierarchical, where the visual scene is initially encoded based on a limited number of feature dimensions detected automatically and in parallel across the visual field. These features, such as colour, shape, and orientation, are characterised independently on a master map of locations, initially specifying where things are but not what they are. The critical point is that incorporating these features into coherent objects relies on focal attention. In the absence of attention, features may combine incorrectly, leading to illusory conjunctions. For example, an optical illusion like the famous “colour square” illusion, where colour and shape are misperceived because of attention lapses, can be described by FIT. The concept highlights how attentional mechanisms play a pivotal role in forming a perception of the visual world and, when compromised, can lead to the experience of optical illusions where features are mistakenly combined, highlighting the role of signs from optical illusions in supporting FIT’s claims about feature incorporation and attention. These examples collectively prove the brain’s complex and sometimes flawed process of interpreting visual information.
Fig 2. The Zollner Illusion (Eneh and Inekwe, 2023).
Fig 3. The Muller-Lyer Illusion (Eneh and Inekwe, 2023).
Brain’s Role in Perception
The brain plays a crucial role in visual perception, with information from the retina undergoing a complex journey through various neural pathways. A study by Tsuchitani (2019) supports the idea that the brain plays a vital role in visual perception. The research states that the original processing site’s retina converts light into neural signals travelling through various brain structures. The optic nerve carries these signals from the retina to the brain. The thalamus’s lateral geniculate nucleus (LGN) is a crucial station where optic nerve fibres terminate and are vital for visual perception. The LGN is organised into layers that process different aspects of vision, such as colour, shape, and movement. The LGN sends signals to the occipital lobe’s primary visual cortex (V1), which is for basic visual processing. Damage to V1 can lead to blindness, which stresses its importance.
Additionally, the importance of the brain’s role in perception becomes even more apparent as visual information branches into two distinct pathways: the dorsal and ventral. These pathways, as described by Ungerleider and Mishkin (1993) and Milner and Goodale (1995), serve different functions in visual perception. The dorsal pathway, frequently called the “where” pathway, is primarily accountable for processing spatial data and guiding actions based on visual input. The ventral pathway, known as the “what” pathway, is involved in identifying and recognising objects and faces. This intricate neural network highlights the brain’s vital role in shaping the perception of the visual world, representing how it orchestrates the flow of visual data to eventually form a conscious perception of the environment.
Fig 4 The flow of Visual information
Fig 4 The flow of Visual information
Neuroplasticity and Perception:
Neuroplasticity is the brain’s ability to reorganise itself by forming new neural connections, and it has a crucial role in shaping perception and sensory processing. Several factors, including learning, familiarity, and the development of new skills, influence this dynamic attribute of the brain. Additionally, neuroplasticity is apparent in the modulation of sensory analysis by higher-order factors such as attention, arousal, and emotion. Proverbio (2022) research highlights the effect of perception and how it is shaped by neuroplasticity that affects brain functioning and can affect how one perceives information. The study suggests early sensory data filtering occurs at the thalamic and amygdala levels. The research findings recommend a more nuanced interaction between early visual processing and later perceptual analysis modulated by top-down factors. Likewise, Mort et al.’s (2003) findings from the study on differential cortical activation during voluntary and reflexive saccades offer compelling support for neuroplasticity in perception. The study validates how distinct cognitive processes, such as the generation of voluntary versus reflexive eye movements, are connected with differential cortical activation patterns. These differences in brain activity suggest that the brain’s neural networks can dynamically adjust and allocate resources based on the specific cognitive task at hand. Also, the study highlights the complex interplay between attention, spatial awareness, and motor control, further emphasising the flexibility of neural pathways in shaping our perceptual experiences. These articles’ findings emphasise the outstanding plasticity of the human brain and its capacity to adapt to changing perceptual demands.
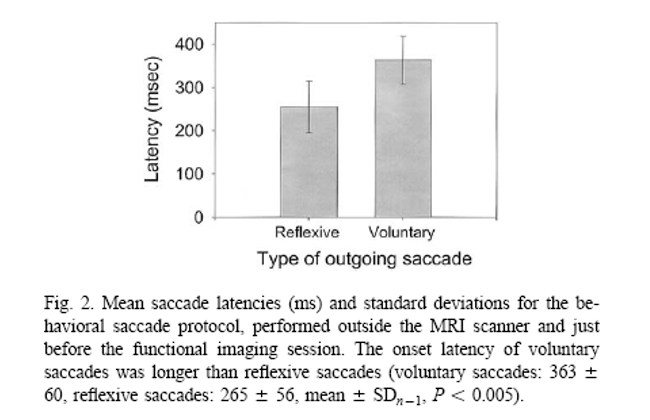
Counterarguments and Rebuttals
The concept that human perception is highly complete and photographic-like, similar to eidetic imagery, is a widespread belief. Marks (2023), a strong supporter of Eidetic, states that Eidetic imagery refers to the capacity to vividly recall images from memory with high precision for a brief history after exposure). Additionally, research by Haber and Haber (1964) evaluated eidetic imagery in elementary school kids. The kids were shown images and then asked to describe what they saw on a blank surface. A small percentage of kids, 8 per cent, exhibited characteristics of eidetic imagery, such as precise recall, cheerful colouration, and visual scanning of the blank space where the image was projected. The study demonstrates that children may exhibit an exceptional capability to recall visual data with great detail, but the prevalence and extent of such eidetic capabilities are limited.
Although some may contend that human perception resembles a highly comprehensive and photographic system, a closer examination reveals the intricacies and constraints of visual awareness. The proof at hand sheds light on these limitations. Firstly, blind spots in retinas supported by the findings of John Wiley and Sons (2011), variations in visual acuity from person to person, and susceptibility to optical illusions all cast doubt on the idea of a perfect photographic perception system. These factors introduce imperfections and idiosyncrasies into visual experience. Research on the role of the brain in perception by Ungerleider and Mishkin (1993) and Milner and Goodale (1995) highlights how brain functioning can significantly influence perception beyond mere sensory input. It highlights that perception is not solely reliant on the raw data our senses receive. Moderately, a complex interaction between sensory input and cerebral processes shapes our understanding of the world around us. Therefore, the idea of a flawless photographic perception system is tested by the inherent limitations of sensory apparatus and the undeniable influence of cerebral processes on perception.
Finally, this essay has explored the complex nature of human visual perception and challenged the conventional trust that our perception offers a comprehensive, almost photographic depiction of the world around us. The limitations of human vision, including blind spots and variations in visual acuity, have been discussed, shedding light on the complexities of our visual awareness. Knowing the fallibility of visual perception can lead to more critical thinking, improved decision-making, and a greater appreciation for the complexities of cognitive processes.
References
Desarkar, P., Rajji, T. K., Ameis, S. H., & Daskalakis, Z. J. (2015). Assessing and Stabilising Aberrant Neuroplasticity in Autism Spectrum Disorder: The Potential Role of Transcranial Magnetic Stimulation. Frontiers in Psychiatry, 6. https://doi.org/10.3389/fpsyt.2015.00124
Encyclopedia.com. (2019). Eidetic Imagery | Encyclopedia.com. Www.encyclopedia.com. https://www.encyclopedia.com/psychology/encyclopedias-almanacs-transcripts-and-maps/eidetic-imagery
Eneh, A.E. and Inekwe, B., (2023). Existence of Spatial-Alignment and Optical Illusions in the Built Environment. INTERNATIONAL JOURNAL OF GENERAL STUDIES (IJGS), 2(3).
Hadders-Algra, M. (2022). Human face and gaze perception is highly context-specific and involves bottom-up and top-down neural processing. Neuroscience & Biobehavioral Reviews, 132, 304–323. https://doi.org/10.1016/j.neubiorev.2021.11.042
John Wiley & Sons. (2011). Appendix A: Human Visual Perception. Practical Image and Video Processing Using MATLAB®, 591–610. https://doi.org/10.1002/9781118093467.app1
Mahabadi, N., & Al Khalili, Y. (2020). Neuroanatomy, Retina. PubMed; StatPearls Publishing. https://www.ncbi.nlm.nih.gov/books/NBK545310/
Marks, D. F. (2023). Phenomenological Studies of Visual Mental Imagery: A Review and Synthesis of Historical Datasets. Vision, 7(4), 67–67. https://doi.org/10.3390/vision7040067
National Eye Institute. (2019, July 16). How the Eyes Work | National Eye Institute. Www.nei.nih.gov. https://www.nei.nih.gov/learn-about-eye-health/healthy-vision/how-eyes-work#:~:text=When%20light%20hits%20the%20retina
Proverbio, A. M. (2022). Perception: A dynamic interplay between genetics and neuroplasticity. Frontiers in Cognition, 1. https://doi.org/10.3389/fcogn.2022.1004576
Tanigawa, H., Majima, K., Takei, R., Kawasaki, K., Sawahata, H., Nakahara, K., Iijima, A., Suzuki, T., Kamitani, Y. and Hasegawa, I., (2022). Decoding distributed oscillatory signals driven by memory and perception in the prefrontal cortex. Cell reports, 39(2).
Tsuchitani, C. (2019). Visual Processing: Cortical Pathways (Section 2, Chapter 15) Neuroscience Online: An Electronic Textbook for the Neurosciences | Department of Neurobiology and Anatomy – The University of Texas Medical School at Houston. Tmc.edu. https://nba.uth.tmc.edu/neuroscience/m/s2/chapter15.html