Introduction
Solvolysis refers to an SN1/SN2 nucleophilic substitution or elimination reaction in which the nucleophile is a solvent. Conventionally, solvolysis of chiral reactants through the SN1 yields a racemate (Chen et al., 2023). Sometimes, the stereochemistry pathway is complex given that the leaving anion remains close to the carbocation, thus shielding it from an attack by the nucleophile (Lanke & Marek, 2020). First reactions can occur when the neighboring groups’ participation with the nonclassical ions takes part as intermediates or transition states. Solvolysis could involve nucleophiles like water, alcohols, acetone, ammonia, alkyl amines, and glucose which consequently determine the name of the process. This experiment examined the rate of nucleophilic substitution in unimolecular solvolysis in a quantitative manner, as depicted in Figure 1, and the factors which affect the reaction rate, which in this case were temperature and solvent.

The experiment utilizes the concept of titrations to observe the kinetics of the solvolysis reaction. The rate law, in this case, defines the speed with which the reaction progresses. Typically, when a reaction occurs, the reactants’ concentration decreases, whereas that of the products increases. Therefore, the reaction rate concerning the reactants will be negative, whereas
it will be positive concerning the products.
The first, ordinarily slow step entails breaking the C-X (carbon-halogen) bond when carrying out the solvolysis process. The carbocation intermediate reacts rapidly with water to form the alcohol and the hydrogen cation (acid) products. Conventionally, the rate-determining step is the slowest; hence in our case, as shown in Figure 2, breaking the carbon-halogen bond will be the rate-determining step which consequently gives the rate law (Fraggedakis et al., 2020). The differentiated rate law becomes as shown in Figure 3, while its integrated form takes the form as depicted in Figure 4:
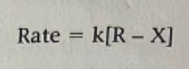
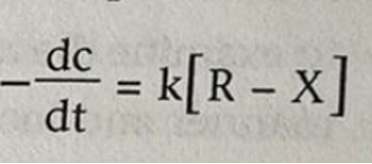
Where: dt is the infinitesimal change in concentration of the
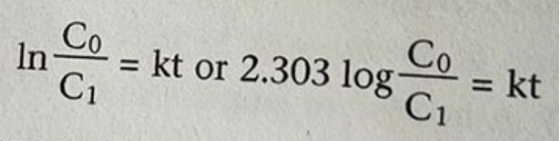
The acetone helps to increase the solubility of t-butyl chloride in water. 10% Sodium hydroxide is used to introduce a base that shows the formation of hydrogen cations (acid). There is also the inclusion of the bromophenol blue indication, whose color changes from blue in the alkaline environment to yellow in acid, showing that the reaction is 10% complete. Therefore, the concentration factor C0/C1 can be expressed in Figure 5 below.
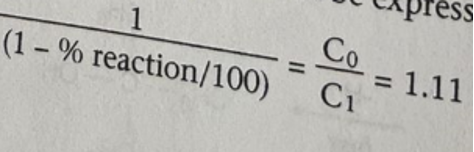
Calculations
Standard method
Given that the amount of alkyl chloride used was 3.0 mL of 0.1 M solution, the number of moles of the alkane will be (3.0 mL × 0.1 M)/1000M = 3.0 × 10-4 mol. Given that the sodium hydroxide was 10% of the mols of the alkyl chloride, the NaOH mol = 0.1 of 3.0 × 10-4 = 3.0 × 10-5 mol.
Discussion
Acetone participates in solvolysis reaction indirectly, given that t-butyl chloride is soluble in acetone (Ernest, 2014.). The acetone polarizes water to yield H+ from water faster, so OH– can interact with the carbocation faster. However, the more polar solvent mixture better stabilizes the developing carbocation. Such a solvent mixture creates a pathway with lower activation energy within its transition state, thus speeding up the reaction rate (He et al., 2023). The 80:20 water: acetone mixture is more polar than a 70:30 mixture, so the solvolysis is faster in the more water-rich solvent mixture. If the solvent mixture were 60% water and 40% acetone, the reaction rate would reduce due to the reduction in the polarity. Water takes pater in the reaction directly since it attacks the carbocation, forming a hydroxonium intermediate, yielding the desired alcohol.
When the reaction was carried out in a hot water bath, the rate constant almost doubled from an average of 0.00168921 sec-1 to 0.02806349 sec-1. When the hot water bath was replaced with a colder one, the rate constant was approximately reduced four times to 0.00041905sec-1. The average kinetic energies of the reactant molecules could explain the changes. As temperature increases, the reacting molecules gain higher average kinetic energy; hence the number of molecules with energy equal to or greater than the activation energy increases (Piskulich et al., 2019). This increases the number of effective collisions and, consequently, the reaction rate.
Conclusion
In this study, the rates of nucleophilic substitution reactions were analyzed by a qualitative analysis considering the factors that affect the reaction rate. The variables, in this case, were hot temperature, cold temperature, and the effect of the solvent. The standard method has an average rate constant of 0.001122676 sec-1 with an average time of 92.67 seconds. When kept in a hot water bath, the average rate constant equaled 0.02806349 sec-1 with an average time of 4.333 seconds. On the other hand, when kept in an ice water bath, the rate constant average equaled 0.00041905 sec-1 with an average time of 254.6667 seconds. The solvent effect had a constant rate average of 0.00279897 sec-1 with an average time of 37.3333 seconds. All in all, this experiment proved that SN1 reactions occur faster when in more polar mixtures. The experiment also revealed that carrying out the reaction in a hot water bath sped the reaction rate and vice versa in the case of a cold water bath.
References
Chen, X., Patel, K., & Marek, I. (2023). Stereospecific nucleophilic substitution at quaternary carbon stereocenters of cyclopropyl carbinols. Chem, 9(2), 266–279.
Ernest Z. (2014). Why does t-butyl chloride undergo solvolysis in 70% water/30% acetone at a rate slower than in 80% water/20% acetone? | Socratic. Socratic.Org. Retrieved April 12, 2023, from https://socratic.org/questions/why-does-t-butyl-chloride-undergoes-solvolysis-in-70-water-30-acetone-at-a-rate-
Fraggedakis, D., Nadkarni, N., Gao, T., Zhou, T., Zhang, Y., Han, Y., Stephens, R. M., Shao-Horn, Y., & Bazant, M. Z. (2020). A scaling law to determine phase morphologies during ion intercalation. Energy & Environmental Science, 13(7), 2142–2152.
He, Z., Lei, Q., Dai, W., & Zhang, H. (2023). Solvent tunes the selectivity of alkenes epoxidation over Ti-Beta Zeolite: A systematic kinetic assessment on elementary steps, kinetically relevant and reaction barriers. Journal of Catalysis, 421, 172–184. https://doi.org/10.1016/j.jcat.2023.03.012
Lanke, V., & Marek, I. (2020). Stereospecific nucleophilic substitution at tertiary and quaternary stereocentres. Chemical Science, 11(35), 9378–9385.
Piskulich, Z. A., Mesele, O. O., & Thompson, W. H. (2019). Activation energies and beyond. The Journal of Physical Chemistry A, 123(33), 7185–7194.